A materials scientist whose revolutionary 3D-printing techniques could make the University of Oregon a worldwide hub in the field is the latest addition to the faculty at the Phil and Penny Knight Campus for Accelerating Scientific Impact.
Paul Dalton, who invented and developed a unique technology enabling high-resolution 3D-printing with the potential to make advanced medical implants, started at the Knight Campus earlier this spring. Dalton’s technology potentially can be applied to any tissue in the body, such as artificial blood vessels and dental implants, and used for bone and tendon repairs, while also supporting nerve regeneration. Several companies have integrated this technique into their 3D bioprinters.“Paul Dalton’s body of work personifies the mission and vision of the Knight Campus in many ways. Over the course of his career, he continues to turn novel scientific discoveries into technologies, products and therapies that benefit people,” said Robert Guldberg, vice president and Robert and Leona DeArmond Executive Director of the Knight Campus. “His expertise has the potential to make the UO and Knight Campus into a global leader in precision 3D printing.”
Dalton, as a researcher at RWTH Aachen University in Germany, began research that led to a 3D printer that works with melted plastics to produce thinly extruded fiber using a process called melt electrowriting. It specializes in making fibers that are as small as 1 micron, which is 1/50 the width of a human hair.
A decade later, while working on materials for use in medicine at Australia’s Queensland University of Technology, Dalton and Dietmar Hutmacher unveiled the first high-resolution 3D printer based on the process.
Dalton had seen that plastics behave differently at varying temperatures, resulting in materials with instabilities when made as small fibers. Turning down the voltage led to improved accuracy and, just before 3D printing emerged, he began to see potential medical benefits that could come with better control of the melted material.
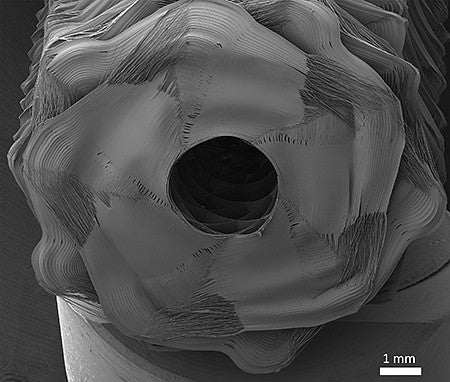
Melt electrowriting is a high-resolution 3D-printing process. In conventional 3D printing, a plastic is melted into a syringe, fed into a printer’s nozzle and deposited continuously in layers. The new technique, Dalton noted, is assisted with high voltages and could lead to tailor-made structures for such things as tissue engineering, textiles and medical implants.“
“We use biomaterials already to solve a whole range of different injuries and diseases,” he said. “There are limits in what we can do now, however, and other products present challenging issues.”
Hernia and prolapse meshes, which medical researchers would like to improve, as well as sutures, are made from molten plastics. Using 3D printing for such materials, Dalton said, could help realize on-demand needs for skin grafts, eye repairs and replacing damaged blood vessels.
Dalton and his international colleagues recently took a big step in both manufacturing precision and quality control by focusing the telescopic lens of a digital camera on the cone and nozzle. The step helped predict fiber size.
The advance was detailed in a proof-of-concept study published online on June 8 in the journal Advanced Materials. For the study, a camera was placed outside the printer, with the lens focused on the nozzle to watch the layered distribution of ultrathin fiber.
“We are making fibers that are 5 microns or 10 microns in size and are soft and flexible, so that’s much smaller than normal 3D printing,” Dalton said. “We have to be very precise because a slight variation of direction of even a couple of microns can change the overall properties. This process allows 350 fiber layers to be printed on top of each other. The previous record for a tube was 10 layers.”
“There are many injuries and diseases that are treated with biomaterials and medical implants,” Dalton said. “We’ve reached a limit in how well we can make them. I am trying to improve the quality of implants, and the research described in this paper lets us watch the manufacturing process in a way that lets us confirm that we’ve made what we intended to make along the way.”
At the Knight Campus, Dalton is working to add more cameras and reduce the printer’s size to that of a standard kitchen coffeepot to make the technology portable.
“We are going to be making different versions of the printer and will incorporate four or five cameras to introduce thermal imaging, high resolution and different angles to allow for a higher volume view of the printing process,” Dalton said. “Eventually, we’d like to have connectivity to a smartphone for monitoring an operating printer.”
"The Knight Campus is an outstanding place to do this research,” he said, “because of the talented people with diverse backgrounds and in diverse disciplines.”
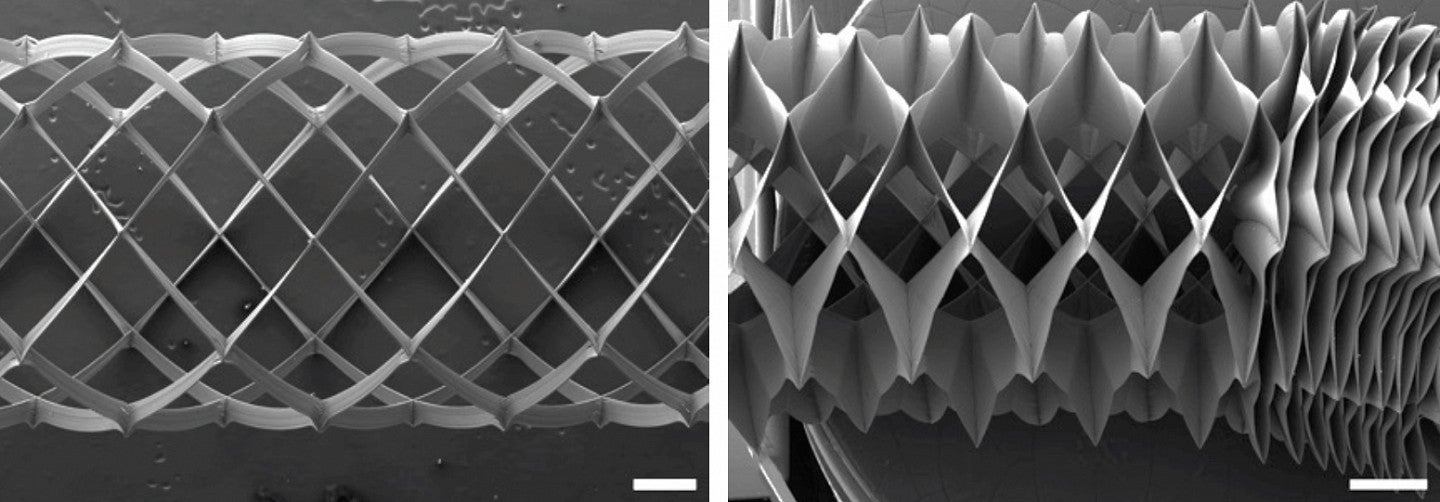
“We can put them on the printers and let them design things that may help them with their work in, say, the humanities, biological arts, computer programming, medicine, the whole spectrum,” he said. “I’m open in what directions we take. I’m looking at how we can make devices for medical uses and advance them into the clinics and beyond.”